MELTIN MMI (hereinafter MELTIN) is a high-tech venture aiming to establish cyborg technology, including artificial bodies and brain-machine interfaces (BMIs), through R&D and commercialization efforts for medical devices, avatar robots, and other items by making the best use of biosignal processing technology and robotics approaches. MELTIN advocates removing physical limitations by means of cyborg technology to create a world in which everyone can maximize their potential for self-realization without constraints due to age or physical condition.
Sharing this corporate vision, we concluded an agreement with MELTIN on financing and joint R&D in October 2018. Since then, we have been collaborating on R&D for sophisticated healthcare devices based on MELTIN’s core technology. Combining MELTIN’s robotics and biosignal processing technology with our long-nourished strengths of findings, knowledge, and experience in the healthcare field, we are conducting a new business development to realize a medical-engineering partnership.
MELTIN’s Unique Technology for Biosignal Analysis
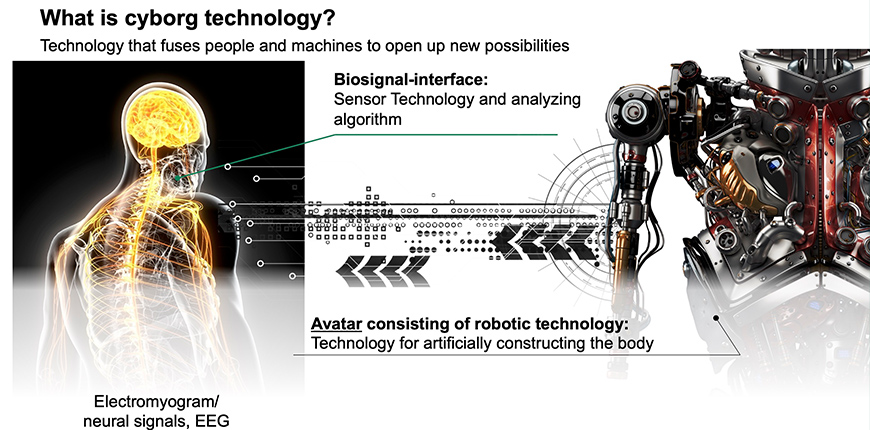
The term “biosignal” refers to an electrical signal that is transmitted through our nerves, in the form of brain waves, electricity in muscle (myoelectricity), and the like. When we move our hand, for example, a signal for “moving the hand” is initiated by commands from the brain, transmitted through the relevant nerves, and reaches the relevant muscles as an electric signal to initiate muscular contractions that move the hand. The electrical signal waveforms and the working muscles they activate differ depending on the intended actions.
MELTIN’s core technology distinguishes among different waveforms and analyzes the motion being made, or intended to be made, by a human body. It carries an algorithm that enables capturing complex biosignals with a large amount of information using a small number of electrodes, and analyzing them at high speed and high accuracy, thus making it possible to convert (reproduce) the signals to highly complex body motions.
Combining its unique biosignal processing technology and robot development, MELTIN aims for developmental achievement in three main areas: body complementation, body augmentation, and body recovery.
Mark Kasuya, CEO of MELTIN, says, “Body complementation is expected to allow a person who has accidentally lost their hand to perform various operations as if they still have a real hand for example, by wearing MELTIN’s robot hand and controlling it through the sensation of their own biosignals, while the robot hand distinguishes among motor intention such as “to grip an object” or “to turn the wrist.”
Regarding body augmentation, the robot hand can be worn as a “third hand” to allow the wearer to perform complex operations that require three hands, using their own biosignals.
As for body recovery, machine-generated, simulated biosignals can be sent to the lower half of the body of a person suffering complete paralysis of the area due to accidental spinal injury, to reactivate the otherwise inert parts of their body.
We believe such complementation, augmentation, and recovery will open a new frontier for what human being has been unable to do so far.”
Restoration of Functional Fingers for Patients with Finger Paralysis
The rehabilitation robot under joint development by MELTIN is designed to facilitate recovery from motor dysfunction. The number of patients with cerebrovascular disorders, major causal diseases of motor dysfunction, was reported to be approximately 1.20 million (*1) in Japan, 7.20 million in the United States (*2), and 13.0 million in China (*3). Cerebrovascular disorders are the leading cause of bedriddenness and the second leading cause of long-term care need in Japan, posing a major concern in our society.
Among the various motor dysfunctions, many patients are suffering from finger paralysis. The proportion of finger paralysis patients who achieve recovery to a practically acceptable level in daily life is lower than in patients with lower limb paralysis (*4), and functional prognosis is poor if grip strength does not improve within 1 month after onset (*5); currently, no effective medical intervention is available for patients in whom finger extension fails to be restored early.
Therefore, patients who cannot expect an early recovery of functional finger motion are unavoidably placed in a situation in which priority is placed on compensatory rehabilitation such as for changing hand dominance without waiting for recovery of the paralyzed hand; the paralyzed digits sometimes remain insufficiently rehabilitated.
On the other hand, recent neuroscience research has shown that changes in plasticity can improve motor function through even in injured brains if appropriate rehabilitation is started early. In this situation, it is expected that rehabilitation will be started early after onset and implemented with increasing frequency and quantity, and that a new approach to neurorehabilitation for improved motor function will be established to reconstruct the cerebral neuronal network by leveraging the plasticity of the tissues of the cranial nervous system.
In fact, therapies are available in which occupational therapists and others repeatedly move the patient’s paralyzed hands and feet to promote functional recovery; however, it is unknown whether the intended motion is made appropriately in severely affected patients, and hence it is unclear whether the treatment is really effective.
Four Features of the Neurorehabilitation Robot
Our neurorehabilitation robot for finger paralysis uses artificial intelligence (AI) to analyze myoelectric signals detected via a patch electrode applied to the forearm, reads the patient’s intention to move their fingers, and drives a hand unit on the palm through a wire.
Even for patients with difficulty in moving their fingers, the robot allows the wearer to “open their fingers” upon wanting and thinking to straighten them, and to “close their fingers” upon wanting and thinking to bend them, aiming to allow the patient to exercise finger motions freely as they intend.
The device is driven in accordance with biosignal then moves the patient's body
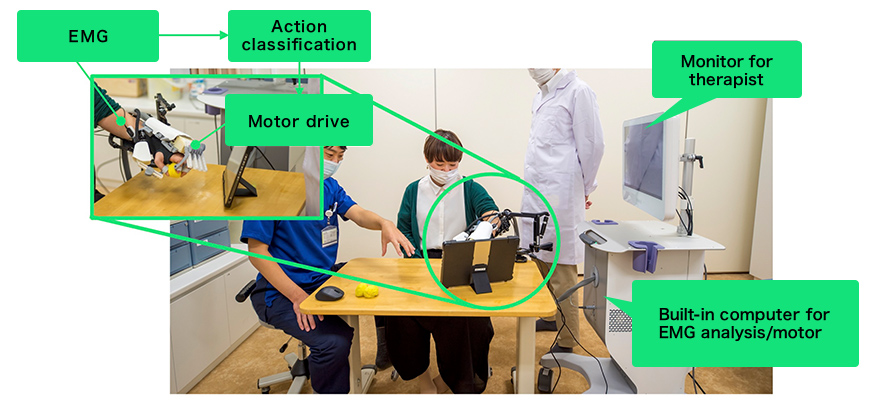
The device is for the most part housed in a cart as shown at the right in the above photo, which also houses a computer for biosignal analysis and a motor for actually driving the fingers.
The device has four main features, as follows:
- 1. Fingers are strongly pulled by wire drive
- Patients with finger paralysis are sometimes affected by spasticity, which necessitate strengthto open the tightly clenched fingers. If the motor is placed near the fingers, the hand unit attachment will be larger and heavier. Conversely, if the weight is reduced, the output power is likely to be insufficient. With these drawbacks in mind, by driving the hand unit via a wire from the motor, rather than by directly driving the hand unit with the motor, we have succeeded in making this device and its attachment extremely lightweight and compact, while securing delicate and powerful assistance. This structure has enabled the robot to support the motions of each of the individual digits with light weight.
- 2. Intent to move identifiable even with weak biosignals (surface myoelectricity)
- In patients with poststroke paralysis, biosignals are often so weak that they are difficult to detect. Even with such weak biosignals, we are working to enable the identification of the user’s intent to move in patients with paralyzed hands by analyzing the signals using an algorithm based on signal amplification technology.
- 3. Algorithm to identify the motions of biosignal “waveforms”
- Most conventional biosignal-based algorithms estimate finger motions according to myoelectric signal intensity. The principle of driving the target in the presence of intense signals and not driving it in the presence of weak signals may serve satisfactorily for estimating the motions of arms and feet, but is unable to identify motions of body parts that move complexly in relation to multiple muscles as in fingers. Therefore, we have developed an algorithm to identify finger motions according to biosignal waveform differences, which, for example, enables an estimation of whether the user is going to present scissors or paper in the scissors-paper-rock game. This new technology is expected to be well applicable to patients with contractures in which intentions to move are difficult to identify based solely on myoelectric signal intensity.
- 4. Robotized parts wearable during occupational therapy
- Our device under development is worn on the back of hand, enabling the user to use their palm freely. Its users are able to grasp and release various objects. In addition, the device allows independent control of the five fingers for holding objects with a broad range of sizes and shapes. This enables the user to practice necessary actions for normal daily life during occupational therapy while wearing the device.
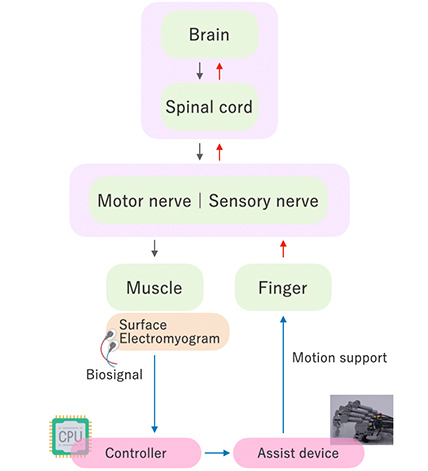
Hence, Sumitomo Dainippon and MELTIN are working together in the clinical rehabilitation field to detect and visualize muscle electricity in paralyzed fingers, and to identify the user’s intent to move from weak myoelectric signals with high accuracy. We are developing a user-friendly neurorehabilitation robot for patients with finger paralysis that can assist their motion with no time lag.
We will join forces to continue developing medical devices and deliver new items to actual clinical settings as early as possible. Our efforts also include compiling evidence in clinical practice and making product improvements such as size reductions of existing models, and we are considering expansion of our market to include home rehabilitation, remote healthcare services, and global business development.
At the Frontier Business Office of Sumitomo Dainippon Pharma Co., Ltd., we are endeavoring to conduct research and development for new value and business by combining our knowledge that has been compiled through R&D activities for pharmaceuticals for psychonurologic diseases with unique technologies and findings of our business partners. We are seeking new partners. If you would like to consider the possibility of collaboration as our business partner, please contact us.
< Data Sources >
- 1)http://www.seikatsusyukanbyo.com/statistics/disease/cerebral-hemorrhage/(WebSite of the Japan Preventive Association of Lifestyle-related Diseases)
- 2)Circulation 2017; 135:e146–603(AHA Report)
- 3)http://j.people.com.cn/n3/2020/0930/c94475-9766118.html(People’s Daily Online Japanese Version / Health and Disease Report on Cardiovascular Diseases in China 2019)
- 4)Heller A, et.al., Arm function after stroke: measurement and recovery over the first three months. J Neurol Neurosurg Psychiatry. 1987; 50: 714–719
- 5)Alan Sunderland 1989 Arm function after stroke. An evaluation of grip strength as a measure of recovery and a prognostic indicator.